Computational Materials Science Center - George Mason University
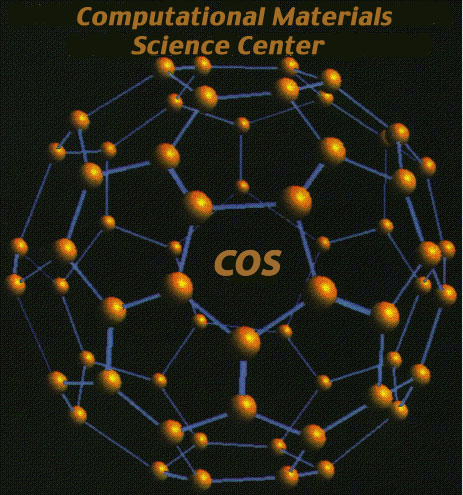 |
Research Interests
The goal of the Center for Simulation and Modeling (CSM) is to develop
new capabilities for simulation of materials using innovative algorithmic
methods for high performance computing. A key component of our industrial
development is the ability to invent and design novel materials and matter in general. Materials
research and materials processing cut across almost every sector of worldwide
industry, from microelectronics to polymers, from pharmaceuticals to gels, from
smart materials such as thermoelectrics to nano-structures, from metals to
ceramics, from magnetic clusters to applications in the recording industry.
More specifically the CSM is aims at the development of the next
generation of atomistic and quantum mechanical modeling tools for the
simulation of matter. A list of subjects under study are: